Nano-optomechanics, light-matter coupling, organic electronics
Reaching the resolution sets by quantum mechanics in sensing is a central goal of present quantum technologies. It will unleash a breadth of possibilities for both fundamental science and applications. In QMBx, we investigate nano-optomechanical systems with potential applications in sensing tiny forces, masses, and electromagnetic fields. For example optically trapped nano-spheres under ultrahigh vacuum are excellent candidates for fundamental macroscopic quantum coherence tests. Other promising topics developed in QMBx are quantum transport in nano-electronic systems acting as qubits or quantum sensors, and molecules in scanning tunnelling microscope forming plasmonic cavities. These systems offer a platform for quantum transduction (the process of converting quantum signals) and for a novel way to fine control chemical reactions. In parallel, coupling multiple quantum degrees of freedom provides new possibilities in hybrid structures (built from different materials), especially in presence of magnetic textures and spin-orbit interactions. Among other research activities, our groups are collaborating to develop new theories for a variety of superconducting hybrid devices, exploiting magnetism and light-matter interaction. Based on our expertise in organic electronics and MEMS, we also design, print, characterize and optimize electronic, optical and mechanical components made either with organic or hybrid materials. Our approach aims to develop printing technologies at low temperature and with low environmental footprint.

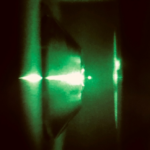

Superconductivity, magnetism, topological phases
Making connection with the material branch of QMBx, we are designing, synthesising and studying new magnetic materials and superconductors with the aim to surpass the quantum computing possibilities offered by the current qubits technologies. Intertwining unconventional superconductivity, magnetism, electronic correlations and topology represent a new paradigm in the field of quantum computing. For example, topological matter is expected to be robust against external perturbations, and thus increase the coherence time while offering new quantum computation schemes. In this context, quantum magnetic materials offer a rich diversity of highly entangled coherent quantum states at a macroscopic scale, with topological phases and quantum spin liquids. The possibility of tuning their properties at molecular and atomic levels is a strong potential source of promising functionalities for future systems and devices.



Cold atoms, artificial matter
Moving to the atomic level, ultra-cold atoms trapped in optical periodic potentials offer a versatile mean to design artificial matter as a quantum simulator. Conventional approaches are however limited to far-field regimes (500 nm atomic distance). Our ambitious goal, supported by trans- laboratory collaborations between experimentalists and theorists, is to lift the technological barriers in order to reach the near-field regime of these quantum simulators, and thus bridge the gap between solid-state physics (0.1 nm) and the 500 nm far-field length scale. This would at long last enable to reach the strongly correlated quantum phases of spin liquids and topological matter in strong synergy with the material branch of QMBx, while taking advantage of the fine-tuned control of optical lattices. Nano-photonic coupling to cold atoms would then be a natural next step as a promising candidate for quantum computing.



Quantum combinatorics, error correction codes
Quantum computation is further supported by a trans-disciplinary working group in computer science and mathematics. This group is expert in quantum combinatorics (quantum chromatic number) applied to distributed quantum computation, and the study of quantum error correction codes is an important research direction in mathematical science in Bordeaux.